Temperature dependence of dielectric nonlinearity of BaTiO3 ceramics
Abstract
In many commercially utilized ferroelectric materials, the motion of domain walls is an important contributor to the functional dielectric and piezoelectric responses. This paper compares the temperature dependence of domain wall motion for BaTiO3 ceramics with different grain sizes, point defect concentrations, and formulations. The grain boundaries act as significant pinning points for domain wall motion such that fine-grained materials show smaller extrinsic contributions to the properties below the Curie temperature and lower residual ferroelectric contributions immediately above the Curie temperature. Oxygen vacancy point defects make a modest change in the extrinsic contributions of undoped BaTiO3 ceramics. In formulated BaTiO3, extrinsic contributions to the dielectric response were suppressed over a wide temperature range. It is believed this is due to a combination of reduced grain size, the existence of a core-shell microstructure, and a reduction in domain wall continuity over the grain boundaries.
Keywords
INTRODUCTION
It is well documented that the dielectric and piezoelectric responses of many ferroelectric materials include contributions from the motion of domain walls, even when the electric field used to excite the material is well below the coercive field[1-6]. This motion of domain walls is important to understand, as it will govern much of the field, time, and frequency dependence of the properties of a ferroelectric material. As an example, domain wall motion contributes substantially to the room temperature permittivity of BaTiO3-based multilayer ceramic capacitors. Similarly, up to 75% of the piezoelectric response of soft PbZr1-xTixO3 ceramics arises from domain wall motion, albeit at the expense of increased hysteresis. The relative extent of domain wall motion for a given material depends explicitly on the history of the sample, the sample microstructure[7,8] and orientation, and the excitation conditions. For example, the following factors are critical to the observed extent of extrinsic contributions to the properties:
Electric field
Higher amplitudes of the AC electric field (EAC0-pk) act to drive domain walls more strongly and can substantially increase the extrinsic contributions to the properties. This increases the relative permittivity and loss tangent with the AC field, which is called dielectric nonlinearity. Often, the increase in relative permittivity is linear with AC electric field, producing the so-called Rayleigh regime (from a modified version of the Rayleigh law originally developed for magnetic materials)[9], i.e.,
where ε' is the relative permittivity, ε'init is the reversible Rayleigh coefficient that includes both the intrinsic response and reversible motion of domain walls or phase boundaries, and α' is the irreversible Rayleigh coefficient[10]. The Rayleigh law is typically observed up to modest electric fields under conditions where the domain structure is unchanged during the measurement and the distribution of the restoring forces for the domain walls is Gaussian[11]. In cases where the domain wall density is changed by the applied field, the Rayleigh law will not be observed, and a more complex formalism, such as first-order reversal curves, needs to be employed[12-16]. In many but not all ferroelectric materials, significant levels of domain nucleation are observed at EAC0-pk exceeding ~a third to a half of the coercive field.
In contrast, because DC biases stabilize the domain state, they also act to suppress extrinsic contributions to the properties. This produces a far stronger DC bias dependence of the relative permittivity[4] than would be predicted based on phenomenological descriptions of the field dependence of the intrinsic permittivity (e.g., intrinsic dielectric stiffening)[17,18]. This progressive loss of the extrinsic contributions to the permittivity is problematic in multilayer ceramic capacitors where the voltage saturation significantly depresses the usable capacitance[19]. In contrast, the suppression is very helpful in some piezoelectric sensing applications, as it increases the figure of merit = piezoelectric coefficient/relative permittivity[20].
Temperature
There is a strong coupling between temperature and extrinsic contributions to the properties. First, domain wall motion is thermally activated such that domain walls become more mobile as temperature increases. Therefore, extrinsic contributions typically rise as the Curie temperature is approached. Other ferroelectric-ferroelectric phase transitions also tend to favor the motion of the mobile interfaces and will induce increases in the extrinsic contributions. Secondly, because domain wall and phase boundary motions are inherently dissipative, substantial levels of extrinsic contributions can increase the sample temperature[21]. Thirdly, changes in temperature can perturb the domain structure and so de-age the material, increasing the extrinsic contributions temporarily.
Time
Domain structures, especially in ceramic materials, are rarely fully stable as a function of time. Thus, over the course of time, the domain structure progressively seeks lower energy configurations. As this occurs, the remaining domain walls tend to be less mobile. This is one of the contributions to the aging of the dielectric or piezoelectric response[22]. Time dependence is also observed in cases where poorly poled piezoelectrics are excited with unipolar waveforms, as is sometimes done in piezoelectric microelectromechanical systems. In this case, the sample poles progressively during use, increasing the remanent piezoelectric coefficient but decreasing the achievable strain during actuation due to progressive loss of the poling strain that is caused by polarization alignment. It is also critical to note that these factors of microstructure, time, temperature, and field are all inextricably linked such that the aging rates will depend on the amplitude of the field used for their characterization[23].
This paper illustrates the temperature dependence of the domain wall contributions to the dielectric properties of BaTiO3 ceramics, with an emphasis on the role of phase transitions, grain size, core-shell microstructures, and point defect concentrations in the response. The resulting temperature dependence of the dielectric response is a complex interplay between these factors.
EXPERIMENTAL PROCEDURE
Samples
Undoped BaTiO3 ceramics were prepared using a BaTiO3 powder (BT02, with a Ba/Ti ratio of 0.996, Sakai Chemical Industry Co., Ltd., Sakai, Japan). To study the effect of grain size, BaTiO3 ceramics with grain sizes of 1.2 µm and 76 µm were prepared. The former was prepared by sintering a BaTiO3 compact at 1,300 °C for 2 h and post-annealing the sintered body at 1,000 °C for 9.5 h[24], while the latter was prepared by sintering the compact at 1,350 °C for 2 h[25]. The grain sizes were measured from the microstructures of polished and chemically etched ceramic surfaces observed by scanning electron microscopy (SEM). Figure 1A and B shows the SEM images of the undoped BaTiO3 ceramics. Two grain lengths were measured for each grain, and the average of these two lengths was calculated for more than 90 grains. The grain size given is the average of the two-length average, and the error bar for the grain size is the standard deviation. The grain sizes were calculated to be 1.2 ± 0.5 µm and 76 ± 37 µm for the BaTiO3 ceramics sintered at 1,300 °C and 1,350 °C, respectively. To study the effect of oxygen partial pressure (pO2) during sintering, BaTiO3 ceramics were sintered at 10-9 atm pO2 at 1,300 °C for 2 h[24]. A microstructure similar to that of the undoped BaTiO3 ceramics sintered at 1,300 °C in air (10-2 atm pO2) was observed by SEM, and the grain size was
Figure 1. SEM images for undoped BaTiO3 ceramics with grain sizes of (A) 1.2 µm and (B) 76 µm and formulated BaTiO3 ceramics sintered at (C) 10-2 atm pO2 and (D) 10-9 atm pO2.
The grain size effect was also studied for model multilayer ceramics capacitors (MLCCs). The size of model MLCCs was 3.2 mm × 1.6 mm × 0.4 mm, and they consisted of ten 7.7-µm-thick BaTiO3-based dielectric layers with X7R specifications and Ni internal electrodes. The grain sizes of the dielectrics were changed from 0.28 ± 0.1 µm, 0.36 ± 0.1µm, and 0.39 ± 0.1 µm by changing the sintering temperature, while the composition of the dielectrics was held constant. The details for the model MLCCs can be found in Refs.[26,27].
Measurement methods
For the undoped and formulated BaTiO3 ceramics, the ac field dependence of the dielectric properties was measured using a lock-in amplifier (SR830, Stanford Research Systems Inc., Sunnyvale, CA, USA), a voltage amplifier, and a charge converter[24]. An ac field frequency of 100 Hz was utilized. The ac field amplitude was increased up to 4 kV/cm; the coercive fields are 4 kV/cm for air-sintered undoped BaTiO3 ceramics with 1.2 µm and 76 µm grain sizes, 3 kV/cm for undoped BaTiO3 ceramics sintered at 10-9 atm pO2 with a 1.2 µm grain size, and 5 kV/cm for formulated BaTiO3 ceramics. The coercive fields were determined from polarization - electric field (P-E) loops measured by a custom-made measurement system at room temperature, as shown in Figure 2.
Figure 2. P-E loops measured at room temperature for (A) undoped BaTiO3 ceramics with a grain size of 1.2 µm and 76 µm, (B) undoped BaTiO3 ceramics sintered at 10-2 atm pO2 and 10-9 atm pO2, (C) formulated BaTiO3 ceramics sintered 10-2 atm pO2 and 10-9 atm pO2, and (D) model MLCCs with various grain sizes. The ac frequency was 10 Hz.
For the model MLCCs, the ac field dependence of the dielectric properties was measured using an LCR meter (4284A, Agilent Technologies Inc., Santa Clara, CA) with a frequency of 1 kHz. The ac field amplitude was increased up to 12-15 kV/cm. The coercive fields of the samples were about 10-15 kV/cm.
The temperature dependence of the dielectric properties was measured upon cooling from 150 °C to
RESULTS AND DISCUSSION
Figure 3 illustrates the temperature dependence of the relative permittivity (ε') (prime) of a BaTiO3 ceramic sample as a function of ac field amplitude. Several points are immediately apparent. First, at temperatures well above the Curie temperature Tc ~125 °C (i.e., measurements at 150 °C), where there should be no ferroelectric domains, there are no domain wall contributions to the properties. As a result, the dielectric permittivity shows very limited dependence on the amplitude of the ac electric field. As the temperature drops to the Curie temperature (data at 125 °C), it is apparent that there is a finite field dependence of ε', which may be due to persistent micropolar regions[28]. Below Tc in the tetragonal ferroelectric regime (data at 80 °C), there is a large population of mobile ferroelectric domain walls in the ceramics, and the nonlinearity in ε' increases. Near any of the phase transitions (apparent as peaks in the permittivity data), both the intrinsic polarizability and the domain wall contributions to the properties rise. The latter is clearly apparent as enhanced dielectric nonlinearity (for example, the data set at 20 °C). Substantial dielectric nonlinearity is observed in the rhombohedral, orthorhombic, and tetragonal ferroelectric phases of BaTiO3. Although not shown here, the domain wall motion contributions to the small signal properties are gradually lost as the temperature approaches 0 K[29].
Figure 3. Temperature and the ac field dependence of the dielectric constant of a BaTiO3 ceramics with a grain size of 1.2 mm. The ac field frequency was 100 Hz. The peaks in the permittivity correspond to the phase transition in BaTiO3; on increasing temperature, the material transforms from a rhombohedral ferroelectric to an orthorhombic ferroelectric to a tetragonal ferroelectric to a paraelectric cubic structure.
The magnitude of the temperature dependence of the extrinsic contributions to the properties is a function of the grain size of the ceramics, as can be seen in comparing the data in Figures 3-5 (Figure 3 uses the same data as Figure 5, and Figure 5 has more data). Figure 4 shows selected data for the dielectric nonlinearity for a large-grained (76 mm average grain size) BaTiO3 ceramics. One consequence of the increased domain wall mobility in large-grained materials is that the electric fields at which the materials transition from Rayleigh-like to non-Rayleigh-like behavior decreases. As a result, Figure 4 shows marked departures from Rayleigh-like behavior at ac field magnitudes under 1 kV/cm in the tetragonal phase field for the BaTiO3. Above these fields, the permittivity first rises very quickly as new domains are nucleated and then goes through a maximum before decreasing as the field amplitude is further increased. This is in contrast to the data in Figures 3 and 5 for finer-grained ceramics, where no marked maximum in the relative permittivity is observed up to 4 kV/cm over the same temperature range.
Figure 4. The ac field dependence of the dielectric properties of undoped BaTiO3 ceramics with a grain size of 76 µm measured at various temperatures.
Figure 5. The ac field dependence of the dielectric properties of undoped BaTiO3 ceramics with a grain size of 1.2 µm, measured at various temperatures.
Figure 5 shows the ac field dependence of the dielectric properties of the undoped BaTiO3 ceramics with a grain size of 1.2 µm measured at various temperatures. It was observed that the ac field dependence was suppressed compared to the large-grained sample over the whole temperature range, as has been reported elsewhere[23]. Grain boundaries are regions where the crystallography of the material is disrupted, and in many cases, these grain boundaries act as pinning centers for the domain walls[25,30-33].
The dielectric data for samples with different grain sizes were quantified by a pseudo-Rayleigh analysis that replaced εinit' with ε'j - the dielectric constant measured at a small field amplitude j. α' was replaced with α'i - the slope for the dielectric constants measured at the field amplitudes j and i (i > j). In this case, pseudo refers to the fact that the permittivity does not increase truly linearly as a function of ac field. Figure 6 shows the temperature dependence of the pseudo-Rayleigh parameters of the BaTiO3 ceramics with a grain size of 1.2 µm and 76 µm. The field i was chosen to ~0.5 kV/cm or ~2 kV/cm to quantify domain wall contributions at subcoercive fields. The temperature dependence of the ε'0.03kV/cm was similar to that of the small signal dielectric constant, except that no dielectric peak was observed at 125 °C for the large-grained ceramics due to its higher Tc of 128 °C[25]. The observation that the ε'0.03kV/cm of the 1.2 µm grain size sample is larger than that of the 76 µm grain size sample below Tc is due to the so-called grain size effect of BaTiO3[7]. For the 76 µm grain size sample, the α'i values were larger than those of the 1.2 µm grain sample due to reduced domain wall pinning at grain boundaries. The α'i values were increased at the rhombohedral to orthorhombic phase transition and orthorhombic to tetragonal phase transition temperatures. In addition, they were increased at 80 °C for the 76 µm grain size sample. This is probably due to a combination of increased domain wall mobility and decreased polarization with increasing temperature. For the 1.2 µm grain size sample, the increases at 80 °C were not observed due to suppressed domain wall mobility. For the 76 µm grain size sample, α'2kV/cm is larger than α'0.5kV/cm above -70 °C, indicating a superlinear ac field dependence. This, in turn, suggests a sublinear response below this temperature, which would be consistent with a restriction in long-range domain wall motion and/or a reduction in the nucleation of new domains.
Figure 6. Temperature dependence of the pseudo-Rayleigh parameters of the BaTiO3 ceramics with grain sizes of 1.2 µm and 76 µm.
Given that domain wall motion is a function of the concentration of pinning sites in the sample, it is also interesting to consider the effect of point defects as potential pinning points. Here, the oxygen vacancy concentration was varied by sintering undoped BaTiO3 ceramics at different oxygen partial pressures, as described elsewhere[24]. Figure 7 shows the ac field dependence of the dielectric properties of BaTiO3 ceramics sintered at 10-9 atm pO2 with a 1.2 µm grain size measured at various temperatures. It was found that the response was similar to that of the sample sintered at 10-2 atm pO2, except that the larger increase in the dielectric properties at moderate ac fields than that of the sample sintered at 10-2 atm pO2 below Tc and larger loss above Tc.
Figure 7. The ac field dependence of the dielectric properties of the undoped BaTiO3 ceramics sintered at 10-9 atm pO2 measured at various temperatures.
The effect of the pO2 on the dielectric nonlinearity was quantified at various temperatures, as shown in Figure 8. Neither sample showed a true Rayleigh regime, presumably due to a combination of defect dipoles associated with oxygen vacancies, residual stresses[34], and pinning from the grain boundaries. It was found that the temperature dependence of the pseudo-Rayleigh parameters was similar, except that the α'i of
Figure 8. Temperature dependence of the pseudo-Rayleigh parameters of the BaTiO3 ceramics sintered at 10-9 atm pO2 and the BaTiO3 ceramics sintered at 10-2 atm pO2 and post-annealed.
In most multilayer ceramic capacitors, the BaTiO3 is formulated to reduce the temperature dependence of the permittivity and increase the reliability when base metal electrodes are utilized[35]. It is thus interesting to compare the temperature dependence of the extrinsic contributions of a formulated dielectric to that of undoped ceramics. Figure 9 shows the ac field dependence of the dielectric properties of formulated BaTiO3 ceramics sintered at 10-2 and 10-9 atm pO2; these samples showed core-shell microstructures[24]. Above Tc, the dielectric constant of both samples was independent of pO2; the permittivities of the two samples were nearly identical in this regime due to the similar grain sizes (both samples had grain sizes of ~0.5 µm) and the absence of a large concentration of mobile interfaces. As expected, provided that the samples are far enough above Tc, there are few residual micropolar regions, and the nonlinear dielectric response of formulated and undoped ceramics is similar. In contrast, below Tc, the irreversible contributions to the permittivity were substantially suppressed in the formulated ceramics relative to the undoped materials. At 20 °C, the ac field dependence of the 10-2 atm pO2 sample was almost linear, while that of the 10-9 atm pO2 sample was sublinear.
Figure 9. The ac field dependence of the dielectric properties of the formulated BaTiO3 ceramics sintered at 10-2 atm pO2 and 10-9 atm pO2 measured at various temperatures.
The dielectric responses were quantified using the pseudo-Rayleigh analysis, as shown in Figure 10. Below Tc, the ε'0.03kV/cm of the 10-2 atm pO2 sample monotonically decreased with temperature, while that of the
Figure 10. Temperature dependence of the pseudo-Rayleigh parameters of formulated BaTiO3 ceramics sintered at 10-2 atm pO2 and 10-9 atm pO2.
It is also notable that the values for α'i for these formulated BaTiO3 ceramics are approximately an order of magnitude smaller than those of the undoped BaTiO3 in the ferroelectric phases. There may be several reasons for this. First, the formulated ceramics had a small grain size of ~0.5 µm, which induced additional grain boundary dilution of the response. Secondly, the formulated BaTiO3 has a lower volume fraction of the ferroelectric phase associated with the introduction of shell regions. These non-ferroelectric regions will also act as capacitors in series that suppress the extrinsic contributions to the response. Thirdly, it is also possible that the core-shell microstructures act to suppress the long coupling of the domain wall motion. It has been shown that domain walls often have some level of continuity across grain boundaries[36] and that the resulting ensembles of domain walls often move as clusters rather than as isolated walls[37,38]. It is not straightforward to separate the relative importance of each of these factors since the second and third, in particular, will also be functions of temperature themselves.
Figure 11 shows the ac field dependence of the dielectric constant for formulated BaTiO3 MLCCs with various grain sizes measured at various temperatures. Above Tc, the dielectric constant was independent of the ac field amplitude. However, the permittivity data do not superimpose for the different grain sizes, presumably because of a difference in the grain boundary dilution of the response[39]. Below Tc, the dielectric constant depends on the ac field, and the ac field dependence increases with decreasing temperature. The ac field dependence was quantified using pseudo-Rayleigh analysis, as shown in Figure 12. The ε'j followed a core/shell response of the dielectric constant. With decreasing grain size, the ε'j decreased. The temperature dependence of the α'i was similar to the formulated BaTiO3 ceramics. The peak at the orthorhombic to rhombohedral phase transition decreased with grain size. This is consistent with the grain size dependence of the undoped BaTiO3 ceramics. It was observed that the grain size dependence of α'i was relatively weak above the Tc of the shell. This result is consistent with the pO2 dependence of α'i for the formulated BaTiO3 ceramics, suggesting the influence of the shell region on irreversible domain wall contributions.
Figure 11. The ac field dependence of the dielectric properties of model MLCCs with different grain sizes measured at various temperatures.
CONCLUSIONS
In conclusion, this paper has demonstrated the temperature dependence of the extrinsic responses in both undoped and formulated BaTiO3. Knowledge of this behavior is important to understand how the materials will perform in working conditions. Given that domain wall motion is thermally activated, it also depends on temperature. The relative magnitude of the extrinsic contributions to the properties is a strong function of the microstructure of the ceramics, especially the grain size and the existence of core-shell microstructures; it is a much weaker function of the concentration of oxygen vacancies.
DECLARATIONS
Acknowledgments
Model MLCC samples were provided from AVX Corporation, Myrtle Beach, SC, USA, for the Center for Dielectrics Studies at Penn State.
Authors’ contributions
The conception and design of the work: Trolier-McKinstry S
The acquisition and analysis of data: Fujii I
The interpretation of data: Fujii I, Trolier-McKinstry S
The writing and revising: Fujii I, Trolier-McKinstry S
Availability of data and materials
The data that support the findings of this study are available from the corresponding author upon reasonable request.
Financial support and sponsorship
The experimental work was funded by the Center for Dielectrics Studies at Penn State. The interpretation of the results was supported by funding from the National Science Foundation and the Center for Dielectrics and Piezoelectrics under Grant No. IIP-1841466 and No. IIP-1841453 and the National Science Foundation grant DMR-2025439.
Conflicts of interest
Both authors declared that there are no conflicts of interest.
Ethical approval and consent to participate
Not applicable.
Consent to participate
Not applicable.
Copyright
© The Author(s) 2023.
REFERENCES
2. Herbiet R, Robels U, Dederichs H, Arlt G. Domain wall and volume contributions to material properties of PZT ceramics. Ferroelectrics 1989;98:107-21.
3. Setter N, Damjanovic D, Eng L, et al. Ferroelectric thin films: review of materials, properties, and applications. J Appl Phys 2006;100:051606.
4. Bassiri-Gharb N, Fujii I, Hong E, Trolier-Mckinstry S, Taylor DV, Damjanovic D. Domain wall contributions to the properties of piezoelectric thin films. J Electroceram 2007;19:49-67.
5. Li S, Cao W, Cross LE. The extrinsic nature of nonlinear behavior observed in lead zirconate titanate ferroelectric ceramic. J Appl Phys 1991;69:7219-24.
6. Otonicar M, Dragomir M, Rojac T. Dynamics of domain walls in ferroelectrics and relaxors. J Am Ceram Soc 2022;105:6479-507.
7. Arlt G. The influence of microstructure on the properties of ferroelectric ceramics. Ferroelectrics 1990;104:217-27.
8. Cao W, Randall CA. Grain size and domain size relations in bulk ceramic ferroelectric materials. J Phys Chem Solids 1996;57:1499-505.
9. Rayleigh L. XXV. Notes on electricity and magnetism. - III. On the behaviour of iron and steel under the operation of feeble magnetic forces. Avaliable from: https://www.tandfonline.com/doi/abs/10.1080/14786448708628000 [Last accessed on 1 Nov 2023].
10. Taylor DV, Damjanovic D. Evidence of domain wall contribution to the dielectric permittivity in PZT thin films at sub-switching fields. J Appl Phys 1997;82:1973-5.
11. Boser O. Statistical theory of hysteresis in ferroelectric materials. J Appl Phys 1987;62:1344-8.
13. Robert G, Damjanovic D, Setter N. Preisach distribution function approach to piezoelectric nonlinearity and hysteresis. J Appl Phys 2001;90:2459-64.
14. Mayergoyz ID. Mathematical models of hysteresis and their applications. Amsterdam: Elsevier Academic Press; 2003.
15. Fujii I, Hong E, Trolier-McKinstry S. Thickness dependence of dielectric nonlinearity of lead zirconate titanate films. IEEE Trans Ultrason Ferroelectr Freq Control 2010;57:1717-23.
16. Stancu A, Ricinschi D, Mitoseriu L, Postolache P, Okuyama M. First-order reversal curves diagrams for the characterization of ferroelectric switching. Appl phys Lett 2003;83:3767-9.
17. Johnson KM. Variation of dielectric constant with voltage in ferroelectrics and its application to parametric devices. J Appl Phys 1962;33:2826-31.
18. Narayanan M, Tong S, Ma B, Liu S, Balachandran U. Modified Johnson model for ferroelectric lead lanthanum zirconate titanate at very high fields and below Curie temperature. Appl phys Lett 2012;100:022907.
19. Tsurumi T, Yamamoto Y, Kakemoto H, Wada S, Chazono H, Kishi H. Dielectric properties of BaTiO3-BaZrO3 ceramics under a high electric field. J Mater Res 2002;17:755-9.
20. Cheng C, Peters T, Dangi A, et al. Improving PMUT receive sensitivity via dc bias and piezoelectric composition. Sensors 2022;22:5614.
21. Hirose S, Aoyagi M, Tomikawa Y, Takahashi S, Uchino K. High power characteristics at antiresonance frequency of piezoelectric transducers. Ultrasonics 1996;34:213-7.
22. Mokrý P, Wang Y, Tagantsev AK, Damjanovic D, Stolichnov I, Setter N. Evidence for dielectric aging due to progressive 180° domain wall pinning in polydomain Pb(Zr0.45Ti0.55)O3 thin films. Phys Rev B 2009;79:054104.
23. Wu K, Schulze WA. Effect of the ac Field level on the aging of the dielectric response in polycrystalline BaTiO3. J Am Ceram Soc 1992;75:3385-9.
24. Fujii I, Ugorek M, Han Y, Trolier-McKinstry S. Effect of oxygen partial pressure during firing on the high AC field response of BaTiO3 dielectrics. J Am Ceram Soc 2010;93:1081-8.
25. Fujii I, Ugorek M, Trolier-McKinstry S. Grain size effect on the dielectric nonlinearity of BaTiO3 ceramics. J Appl Phys 2010;107:104116.
26. Nies C, Berolini M. Factors in improved DC bias performance in X7R capacitors. In: The 12th US-Japan seminar on dielectric piezoelectric ceramics, 2005 Nov 6-9; Annapolis, USA.
27. Fujii I, Trolier-McKinstry S, Nies C. Effect of grain size on dielectric nonlinearity in model BaTiO3-based multilayer ceramic capacitors. J Am Ceram Soc 2011;94:194-9.
28. Garten LM, Lam P, Harris D, Maria JP, Trolier-McKinstry S. Residual ferroelectricity in barium strontium titanate thin film tunable dielectrics. J Appl Phys 2014;116:044104.
29. Zhang QM, Wang H, Kim N, Cross LE. Direct evaluation of domain-wall and intrinsic contributions to the dielectric and piezoelectric response and their temperature dependence on lead zirconate-titanate ceramics. J Appl Phys 1994;75:454-9.
30. Randall CA, Kim N, Kucera JP, Cao W, Shrout TR. Intrinsic and extrinsic size effects in fine-grained morphotropic-phase-boundary lead zirconate titanate ceramics. J Am Cera Soc 1998;81:677-88.
31. Ghosh D, Sakata A, Carter J, et al. Domain wall displacement is the origin of superior permittivity and piezoelectricity in BaTiO3 at intermediate grain sizes. Adv Funct Mater 2014;24:885-96.
32. Marincel DM, Zhang H, Jesse S, et al. Domain wall motion across various grain boundaries in ferroelectric thin films. J Am Ceram Soc 2015;98:1848-57.
33. Hennessey G, Peters T, Tipsawat P, Checa M, Collins L, Trolier-McKinstry S. Domain wall motion across microstructural features in polycrystalline ferroelectric films. Acta Mater 2023;250:118871.
34. Demartin M, Damjanovic D. Dependence of the direct piezoelectric effect in coarse and fine grain barium titanate ceramics on dynamic and static pressure. Appl phys Lett 1996;68:3046-8.
35. Kishi H, Mizuno Y, Chazono H. Base-metal electrode-multilayer ceramic capacitors: past, present and future perspectives. Jpn J Appl Phys 2003;42:1-15.
36. Mantri S, Daniels JE. Ferroelectric domain continuity over grain boundaries for tetragonal, orthorhombic, and rhombohedral crystal symmetries. IEEE Trans Ultrason Ferroelectr Freq Control 2018;65:1517-24.
37. Bintachitt P, Jesse S, Damjanovic D, et al. Collective dynamics underpins Rayleigh behavior in disordered polycrystalline ferroelectrics. Proc Natl Acad Sci USA 2010;107:7219-24.
38. Peters T, Zhu W, Checa M, Collins L, Trolier-McKinstry S. Influence of doping and thickness on domain avalanches in lead zirconate titanate thin films. Appl Phys Lett 2023;122:132906.
Cite This Article
Export citation file: BibTeX | RIS
OAE Style
Fujii I, Trolier-McKinstry S. Temperature dependence of dielectric nonlinearity of BaTiO3 ceramics. Microstructures 2023;3:2023045. http://dx.doi.org/10.20517/microstructures.2023.43
AMA Style
Fujii I, Trolier-McKinstry S. Temperature dependence of dielectric nonlinearity of BaTiO3 ceramics. Microstructures. 2023; 3(4): 2023045. http://dx.doi.org/10.20517/microstructures.2023.43
Chicago/Turabian Style
Fujii, Ichiro, Susan Trolier-McKinstry. 2023. "Temperature dependence of dielectric nonlinearity of BaTiO3 ceramics" Microstructures. 3, no.4: 2023045. http://dx.doi.org/10.20517/microstructures.2023.43
ACS Style
Fujii, I.; Trolier-McKinstry S. Temperature dependence of dielectric nonlinearity of BaTiO3 ceramics. Microstructures. 2023, 3, 2023045. http://dx.doi.org/10.20517/microstructures.2023.43
About This Article
Special Issue
Copyright
Data & Comments
Data
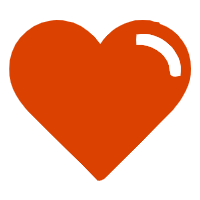

Comments
Comments must be written in English. Spam, offensive content, impersonation, and private information will not be permitted. If any comment is reported and identified as inappropriate content by OAE staff, the comment will be removed without notice. If you have any queries or need any help, please contact us at support@oaepublish.com.